
Most people understand that earthquakes can produce catastrophic damage to the built environment. However, given that large earthquakes are relatively rare, and that the television news cameras typically move on a few days after any disaster, a lot of people’s understanding of the effects of earthquakes may be shaped more by bad disaster movies than reality.
This is unfortunate because, as with other natural hazards, there are public policy choices regarding earthquake risk and recovery that would benefit from an informed public. Among these choices are building code requirements for earthquake-resistant construction. While it may violate some people’s idea of “common sense”, earthquake-resistant structural design is required by code to some extent in all jurisdictions in the United States. Another controversy is mandatory seismic retrofit requirements in some west coast cities for non-ductile concrete and “soft-story” wood frame buildings. Since news organizations may have little more scientific literacy than the public they must inform, they may have too simple an understanding of earthquakes and may overstate associated risks. This could result in disaster mitigation resources being spread too thin.
Whether you live in the west, the east or the heartland, you are exposed to some earthquake risk. It is therefore important to know certain things about earthquakes and how structures resist them.
Earthquakes can happen anywhere.
People generally associate earthquakes with places like California and Alaska, where well-known faults along tectonic plate boundaries have produced large earthquakes throughout recorded history. However, there are seismic hot spots that are far from the plate boundaries. Major earthquakes have occurred in places like New Madrid, Missouri, Yellowstone National Park, Charleston, South Carolina and probably New Hampshire.
Earthquakes within the plate interiors are not always well understood. In places like Hawaii, vulcanism causes earthquakes. Wastewater injection by the oil and gas industry has been found to induce minor earthquakes in Oklahoma. However, more commonly the specific cause of a given intraplate earthquake is unknown, since they typically do not occur at known active faults. Intraplate earthquakes are therefore even more unpredictable than earthquakes along the plate boundaries. Some seismically active intraplate locations appear to represent aftershock sequences for large historical earthquakes. That means that the next severe earthquake could happen somewhere else, where there is not a history of seismic activity. So even if you do not live in a seismically active region, you are exposed to some, perhaps small risk a severe earthquake. The seismic hazard models and building codes account for this and require some level of earthquake resistant design in all 50 states.
There is a lot we do not know.
By the 1960s or 1970s, faith in scientific research and theories had a lot of people believing that the ability to predict earthquakes was right around the corner. I once read a pamphlet from that era claiming to be able to explain earthquakes in New England as being the product of particular geologic feature. The pamphlet was used to justify the safety of expanding a nuclear power plant, since the plant was some distance away from the purportedly causative features.
The theory posited by the pamphlet has been largely discredited. The ability to predict earthquakes, even at known major faults is still mostly limited considering how much a fault moves compared to how much it needs to move to avoid storing energy that could be later released as an earthquake. Forecasts are therefore typically statistical, based on the assumption that earthquakes in the future will more likely occur where earthquakes have occurred in the past and that smaller earthquakes are more common than larger ones. This approach relies on cataloging historic earthquakes, which can be difficult since complete records may only be available from the mid-twentieth century onward, or a couple hundred years in the case of large earthquakes, which is not long in geologic time.
The earthquake forecast is essentially the product of several statistical models. The first model predicts how often a source area will produce an earthquake of a given size. Since the seismic hazard at a particular site is produced by earthquakes of varying size and source location, a model is required to estimate the ground motion at a given location as a function of magnitude, distance and sometimes other attributes of each earthquake that might be felt at the site. Other models might be required to convert parameters so different data sets can be combined or to model how a soil and rock at a site change the ground motion. Whenever multiple models are coupled in the same analysis, uncertainties can be compounded.
Size isn’t everything.
When an earthquake occurs, the media often reports the magnitude on the “Richter Scale”. The Richter magnitude scale is one of the less useful of several scales used to quantify the energy released or the amplitude of the ground motion produced by an earthquake. However, the scales are somewhat similar: the magnitude is the exponent of a particular measured quantity. So a magnitude of 5.0 reflects a measurement ten times larger than that of a magnitude 4.0. The ground motion produced by the earthquake diminishes with distance from the source, however, so knowing the magnitude does not adequately describe how the earthquake will be experienced by people and structures.
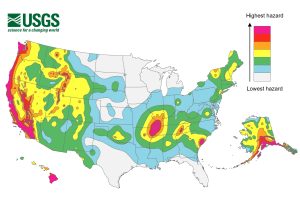
Earthquake ground motion consists of waves, oriented in different directions and at different frequencies, all superimposed on each other. Different materials respond differently to each wave, amplifying some and attenuating others. For example, at the plate boundaries, the crust is more fractured and warmer than within the plate interiors. Therefore the crust is softer and dissipates the energy from ground motion within a shorter distance. That is why west coast earthquakes are not felt as far from their sources as east coast earthquakes. More locally, the soil conditions and structures can amplify or attenuate certain frequencies of the ground motion.
Engineers have to consider which of the waves a structure will amplify. If the structure amplifies frequencies in the ground motion with high amplitudes, then the force effects and risk of collapse will be greater. However, if the structure responds to different frequencies that have lower amplitudes, it will ‘feel’ the earthquake less severely and be safer for occupants. Therefore engineers are more concerned with certain ‘spectral’ values, usually accelerations, that define the critical frequencies of vibration.
There is no such thing as an earthquake-proof structure.
Given the difficulty of predicting earthquakes, it is not practical to design structure to survive any and all earthquakes. There is always some risk that a structural will experience larger ground motions than what was used as the basis for design. Thus there is no such thing as an absolutely earthquake-proof structure. However, even with the difficulty of quantifying earthquakes and the response of structures to earthquake effects, there is a lot that structural engineers know about making buildings safer during earthquakes.
The typical approach to earthquake-resistant design is to detail the structure to avoid collapse during an earthquake with a probability of being exceeded of just a few percent during the structure’s design life. Structures are designed to absorb energy through damage, while remaining stable, not unlike automobiles are designed to absorb energy in crashes while protecting the occupants. The mass, strength and stiffness of the materials are controlled so that the structure’s response will be more predictable. Brittle materials and less reliable details are avoided or relegated to non-critical locations. In the case of the rare, design event, occupants should be able to safely evacuate. This approach balances construction cost with the risks to people and property and is appropriate for most common buildings. However, some structures must be usable immediately after a large earthquake and will be designed to sustain more limited damage, at a higher construction cost.
It is also known that certain types of structures perform better during earthquakes. For example, conventionally constructed dwellings typically perform well because they are lightweight, highly redundant and usually non-brittle. For most people, the risk of falling objects might be the greatest earthquake risk in the home. (This is why you should anchor your bookcases to the wall.) Small, single-span bridges also perform well because they more-or-less go along for the ride and do not develop large forces. Both types of structures are exempt from some of the more burdensome earthquake-resistant design requirements, at least outside high-hazard regions. You may find it surprising that tall buildings can perform well. Tall buildings amplify frequencies that are smaller than those common with earthquake motions. Instead, they are more susceptible to oscillating in the wind and must be designed accordingly. It always annoys me that disaster movies often show skyscrapers splintering and collapsing during “the big one”. While less dramatic on-screen, partial collapses of older masonry and concrete buildings would be much more likely in a large earthquake.
As is the case with other hazards, structures are now built to be safer during earthquakes due to learning from past failures and implementing this experience into construction codes. This has made the codes more difficult to use for professionals and has made increased the cost of construction for builders and owners. This represents a public policy decision that the lives saved from this approach are worth the added cost. But there are still choices to be made: Do we mitigate older structures? How do we resolve competing priorities of hazard mitigation and historic preservation? Under what circumstances does the economic and social cost of disaster recovery justify even more stringent codes and standards? These questions are analogous to those surrounding other natural and man-made hazards to structures. An informed public and policy-makers are crucial to attaining better outcomes. Everyone needs to know more than they can learn from watching disaster movies.